Introduction
Acid-base indicators are substances that change color in response to changes in pH, making them essential in titration experiments to determine the equivalence point. Several theories explain how these indicators function, including Ostwald’s Ionization Theory, Quinonoid Theory, and the Modern pH Theory. Each of these theories provides insights into the mechanism by which indicators exhibit color changes in different pH environments.
1. Ostwald’s Ionization Theory
This theory, proposed by Wilhelm Ostwald, is based on the principle that acid-base indicators are weak acids or weak bases that partially ionize in solution. The ionized and unionized forms of the indicator have distinct colors. According to this theory:
- An acid-base indicator exists in equilibrium between its ionized and non-ionized forms.
- The color of the solution depends on the relative concentration of these two forms.
- When the pH of the solution changes, the equilibrium shifts, causing a visible color change.
For example, phenolphthalein is colorless in acidic conditions but turns pink in alkaline solutions due to the ionization of its molecular form.
Advantages of Ostwald’s Ionization Theory
- Explains Electrolytic Conductivity: The theory effectively explains why electrolytes conduct electricity by ionizing in solution.
- Degree of Ionization: It introduces the concept of the degree of ionization, which helps predict the strength of acids and bases.
- Dissociation Constant (Ka and Kb): Ostwald’s dilution law quantitatively relates the ionization of weak electrolytes to their concentration, helping in calculations.
- Correlation with Colligative Properties: It explains the deviations in colligative properties like osmotic pressure, boiling point elevation, and freezing point depression due to ion formation.
- Supports Neutralization Reactions: The theory supports the idea that acid-base neutralization occurs due to the reaction between H⁺ and OH⁻ ions to form water.
Disadvantages of Ostwald’s Ionization Theory
- Fails for Strong Electrolytes: It assumes that electrolytes ionize only partially, which does not apply to strong electrolytes like NaCl and HCl, which are almost completely ionized in solution.
- Ignores Inter-Ionic Attractions: It does not consider the interactions between ions in highly concentrated solutions, leading to deviations from the expected behavior.
- Limited to Aqueous Solutions: The theory primarily applies to aqueous solutions and does not explain ionization in non-aqueous solvents.
- Does Not Explain Ion Pair Formation: In highly concentrated solutions, some ions form pairs rather than remaining free, which the theory does not account for.
- Inapplicable to Non-Electrolytes: The theory does not explain the behavior of substances that dissolve in water but do not ionize, such as sugar and alcohol.
2. Quinonoid Theory
The Quinonoid Theory suggests that acid-base indicators undergo structural transformations between two distinct forms: the benzenoid form and the quinonoid form. Each structural form absorbs light differently, resulting in different colors. Key points of this theory include:
- The benzenoid form is stable in one pH range, while the quinonoid form is stable in another.
- A shift in pH causes a transition between these forms, leading to a color change.
- The transformation is influenced by the nature of the indicator and the surrounding medium.
For instance, methyl orange exhibits a structural change between red (acidic form) and yellow (basic form), demonstrating the quinonoid transition.
Advantages of Quinonoid Theory
- Explains Color Changes in Indicators: The theory successfully explains how acid-base indicators undergo structural changes, leading to color shifts in different pH environments.
- Supports the Reversibility of Indicators: It justifies why indicators like phenolphthalein and methyl orange exhibit reversible color changes depending on the pH.
- Relates Structure to pH Sensitivity: The theory explains how the transition between the benzenoid and quinonoid forms depends on protonation or deprotonation.
- Widely Applicable to Organic Indicators: Many acid-base indicators follow this mechanism, making it a valuable concept in titration and analytical chemistry.
Disadvantages of Quinonoid Theory
- Fails for Some Indicators: Not all indicators follow the quinonoid mechanism, as some exhibit color changes due to other structural modifications.
- Does Not Explain Fluorescence and Absorption Variations: Some indicators exhibit fluorescence or changes in UV absorption that are not directly related to the benzenoid-quinonoid transition.
- Limited to Certain Classes of Compounds: This theory primarily applies to organic indicators and does not generalize to all pH-sensitive compounds.
- Does Not Account for Solvent Effects: This theory does not adequately explain how different solvents influence the color changes of indicators.
3. Modern pH Theory
This theory is based on the Henderson-Hasselbalch equation, which provides a mathematical relationship between the pH of the solution and the ratio of acidic and basic forms of the indicator. According to this theory:
- The proportion of its acidic and basic species determines the color of the indicator.
- The pH at which the indicator changes color is close to its pKa value.
- The color change occurs over a small pH range, known as the transition range.
The equation used is:
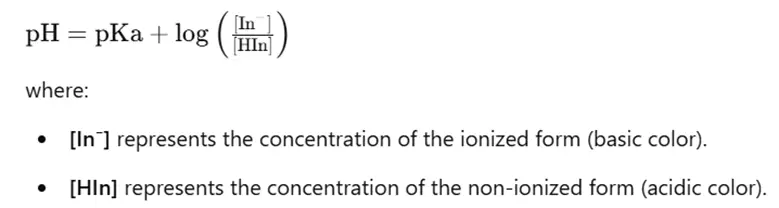
This theory accurately predicts the pH range at which an indicator changes color and plays a crucial role in modern analytical chemistry.
Advantages of Modern pH Theory
- Universal Applicability: The theory applies to all solvents, not just water, allowing a broader understanding of acid-base behavior in various chemical environments.
- Accurate Quantification: The use of the pH scale provides precise numerical values for acidity and basicity, improving measurements in chemistry, biology, and industry.
- Explains Acid-Base Behavior in Non-Aqueous Systems: Unlike Arrhenius and Brønsted-Lowry theories, modern pH theory extends to solvents like ammonia and acetic acid.
- Supports Industrial and Biological Applications: pH control is crucial in pharmaceuticals, food science, medicine, and environmental monitoring, making the theory highly practical.
- Improved Understanding of Acid-Base Reactions: It incorporates concepts like buffer solutions, pKa values, and acid dissociation constants for better chemical analysis.
Disadvantages of Modern pH Theory
- Dependent on Reference Electrodes: The measurement of pH requires calibration and reference standards, making precise determination dependent on external conditions.
- Limited at Extremely High or Low pH: The accuracy of pH measurements decreases in very acidic or basic conditions due to electrode instability and ion interference.
- Temperature and Ionic Strength Sensitivity: Variations in temperature and ionic strength affect pH measurements, requiring corrections in different experimental setups.
- Inapplicable to Non-Proton Systems: The modern pH concept relies on hydrogen ion activity, limiting its use in non-protonic acid-base reactions (e.g., Lewis acid-base interactions).
- Instrumentation Challenges: Accurate pH determination requires specialized equipment like pH meters and electrodes, which may be costly and require maintenance.
Conclusion
Understanding the theories of acid-base indicators is crucial for selecting the appropriate indicator for titrations. Ostwald’s Ionization Theory explains color change through ionization equilibrium, Quinonoid Theory attributes it to structural transformations, and the Modern pH Theory provides a quantitative approach using the Henderson-Hasselbalch equation. Each theory contributes to a deeper understanding of how indicators function, ensuring precision in acid-base titration experiments.